Congrats to the 2019-20 Department of Physics Scholarship & Award Winners! Please join us in recognizing their achievements at a virual ceremony to be held via Blackboard Collaborate on Thursday, May 7, at 5pm CDT.
Month: April 2020
2020 Commencement
Please join us for a live virtual Commencement ceremony for undergraduate Physics and AMEP majors and Physics PhDs. For more info and a list of graduates, please visit https://www.physics.wisc.edu/commencement-2020/
Physics Department featured in UW article on virtual lab course instruction
It’s one thing to have to move a lecture course online, but lab courses — where students learn by doing — is an entirely different story. University Communications spoke to instructors in Physics, including Prof. Deniz Yavuz and Dr. Jim Reardon, about the transition to online lab instruction.
Manipulating the magnetic response to light in natural materials
When light moves from one material into another, it bends — like how a partially submerged object appears distorted under water when viewed from above. What if, instead of bending, a material could change the light so much that the material was no longer visible at all?
In a study published in Physical Review A, University of Wisconsin–Madison researchers have shown for the first time that a similar response can be obtained and manipulated in naturally-occurring materials. The findings have implications from the development of “perfect” lenses for improved microscopy to Harry Potter-esque invisibility cloaks.
Visible light is made of both magnetic and electric fields, and the refractive index of a material — how much it bends the light — is determined by how the material interacts with those two fields. Nearly all materials we encounter in everyday life, though, interact entirely with light’s electric field.
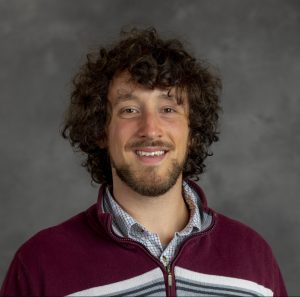
Researchers have spent the past two decades developing artificial materials that more strongly interact with light’s magnetic field by manipulating the refractive index. With a strong enough response, the material could eventually have a negative refractive index, leading to unique optical properties. However, the response in synthetic materials is limited by the size of their repeating units. A naturally-occurring crystal that has much smaller unit cells is likely a better choice.
“Part of producing a negative refractive index is that the material needs to have a strong response to both electric and magnetic fields, so the big challenge is getting that magnetic response in natural materials,” explains Zach Buckholtz, a graduate student in UW–Madison physics professor Deniz Yavuz’s group and lead author of the study. “A few years ago, we published a paper showing that the crystal we’re working with has a magnetic response, and in this study, we were able to manipulate the response.”
The natural material Buckholtz is working with is a silicon-based crystal, which in general is optically ordinary, except that it has been “doped” with the rare earth metal Europium. Rare earth metals are unique in that they contain an abundance of electrons in the atoms’ outer energy shells. Those electrons can then work together to create a bigger magnetic response, but only if they are all in tune with each other.
“If you have some magnetic response and a much larger electric response to light, you can connect those two responses,” Buckholtz says. “To get to a negative refractive index from there, you have to set up coherences between the energy levels, meaning you have to make sure all those energy levels are oscillating together.”
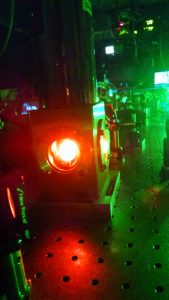
To show they can manipulate the magnetic response, Buckholtz and Yavuz did two things. First, because the crystal is a mix of ions with slightly different electron responses, they needed to set up their experimental system to select for one class of ion. This uniformity allows for a cleaner interpretation of the results.
“We send a laser into the crystal, and then measure how much of the light is transmitted. But because the crystal isn’t perfect, instead of seeing a narrow peak for the transitions, you’ll see a really broad transition,” Buckholtz explains. “So, we do this procedure known as spectral hole burning to clear out the ions we don’t want and then we’ll be left with just one transition, which is necessary to move on to experiments that involve coherence.”
Next, they wanted to show if they could increase the magnetic response. To do so, they needed to take those selected ions, put them in coherence, and then measure the response compared to ions not in coherence. In these experiments, they shined one (a probe beam) or two (probe and coupling beam) wavelengths of laser at the ions. Both lasers excite electrons in the ions to a higher-energy state, and the scientists can again measure how might light is transmitted through the crystal as a readout of the electron transitions.
“With just the probe beam, we see just the normal transition, and that’s what we did in our previous study. But with the coupling beam added in, it connects and adds another transition state in there,” Buckholtz says. “If those states are in coherence, they cancel each other out, and we see that effect as a peak in transmitted light, which means the index of refraction is going toward zero.”
Buckholtz notes that the magnetic response they see is not yet large enough to produce the materials with interesting new optical properties they are hoping for. Still, he says, this work provides a path forward to continue manipulations to improve the response, such as investigating different rare earth metals.
“We have a magnetic response, we can set up coherence, and we can manipulate the response,” Buckholtz says. “Now, we want to increase the scale of the response to with a goal of eventually making the refractive index below zero.”
Saffman group part of team awarded $7.4M grant to apply quantum computers to real-world problems
Wisconsin Quantum Institute director and professor of physics Mark Saffman and his research group are part of a team that will attempt to make quantum computing hardware more applicable to real-world problems.
The up to $7.4 million Defense Advanced Research Projects Agency (DARPA) funding is through the ONISQ program — Optimization with Noisy Intermediate-Scale Quantum devices. ColdQuanta is the primary recipient of the funding, and Saffman’s group at the University of Wisconsin–Madison, along with a national lab and other universities, are partners.
“We’re in this era of development of quantum computing hardware that has been termed NISQ, and that’s because we don’t have error correction running on our quantum hardware,” says Saffman, who is also a UW–Madison professor of physics and chief scientist for quantum information at ColdQuanta. “The question is, can we do anything useful with this? Because the outlook for having a real error-corrected quantum computer that you could run very long calculations still seems to be a long way away, but we have these NISQ machines today, and they’re getting better all the time.”
New research helps explain why the solar wind is hotter than expected
When a fire extinguisher is opened, the compressed carbon dioxide forms ice crystals around the nozzle, providing a visual example of the physics principle that gases and plasmas cool as they expand. When our sun expels plasma in the form of solar wind, the wind also cools as it expands through space — but not nearly as much as the laws of physics would predict.
In a study published April 14 in the Proceedings of the National Academy of Sciences, University of Wisconsin–Madison physicists provide an explanation for the discrepancy in solar wind temperature. Their findings suggest ways to study solar wind phenomena in research labs and learn about solar wind properties in other star systems.
“People have been studying the solar wind since its discovery in 1959, but there are many important properties of this plasma which are still not well understood,” says Stas Boldyrev, professor of physics and lead author of the study. “Initially, researchers thought the solar wind has to cool down very rapidly as it expands from the sun, but satellite measurements show that as it reaches the Earth, its temperature is 10 times larger than expected. So, a fundamental question is: Why doesn’t it cool down?”
Physicists to improve plasma fusion mirror devices with $5 million grant
University of Wisconsin–Madison plasma physicists will harness the power of high-temperature superconducting magnets to design and build a more efficient plasma fusion device, thanks to a two-year, $5 million U.S. Department of Energy grant awarded April 7.
The team, led by physics Professor Cary Forest, has been conducting fusion research for over two decades and expects this new device — the Wisconsin HTS Axisymmetric Mirror (WHAM) — will serve as a prototype for the next generation of fusion reactors.
“Neutrons generated from fusion are useful for many things, from making medical isotopes to potentially being a power source in the future,” Forest says. “Our idea initially — and this was funded by a UW2020 grant — was to build a neutron source which could go several orders of magnitude beyond current medical isotope production efficiencies but also provide a key first step in the direction of advancing fusion energy.”
The Milky Way’s satellites help reveal link between dark matter halos and galaxy formation
This story was adapted from one originally published by Nathan Collins at SLAC. Read the original version here.
Just as the sun has planets and the planets have moons, our galaxy has satellite galaxies, and some of those might have smaller satellite galaxies of their own. To wit, the Large Magellanic Cloud (LMC), a relatively large satellite galaxy visible from the southern hemisphere, is thought to have brought at least six of its own satellite galaxies with it when it first approached the Milky Way, based on recent measurements from the European Space Agency’s Gaia mission.
Astrophysicists believe that dark matter is responsible for much of that structure, and now researchers with the Dark Energy Survey — including University of Wisconsin–Madison assistant professor of physics Keith Bechtol and his research group — have drawn on observations of faint galaxies around the Milky Way to place tighter constraints on the connection between the size and structure of galaxies and the dark matter halos that surround them. At the same time, they have found more evidence for the existence of LMC satellite galaxies and made a new prediction: If the scientists’ models are correct, the Milky Way should have an additional 150 or more very faint satellite galaxies awaiting discovery by next-generation projects such as the Vera C. Rubin Observatory’s Legacy Survey of Space and Time.
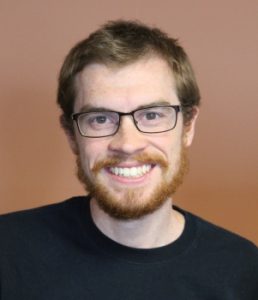
Two new studies, forthcoming in the Astrophysical Journal and available as preprints (pre-print 1; pre-print 2), are part of a larger effort to understand how dark matter works on scales smaller than our galaxy.
“The ultra-faint galaxies that orbit the Milky Way are small clouds of dark matter with just enough stars to see that they exist. They are nearly invisible, but if spotted, they make excellent natural laboratories to study dark matter,” Bechtol says. “We hope to learn what dark matter is made of, how it was produced in the early Universe, and what relationship it has to the known particle species.”
Shining galaxies’ light on dark matter
Astronomers have long known the Milky Way has satellite galaxies, including the Large Magellanic Cloud, which can be seen by the naked eye from the southern hemisphere, but the number was thought to be around just a dozen or so until around the year 2000. Since then, the number of observed satellite galaxies has risen dramatically. Thanks to the Sloan Digital Sky Survey and more recent discoveries by projects including the Dark Energy Survey (DES), the number of known satellite galaxies has climbed to about 60.
Such discoveries are always exciting, but what’s perhaps most exciting is what the data could tell us about the cosmos. “For the first time, we can look for these satellite galaxies across about three-quarters of the sky, and that’s really important to several different ways of learning about dark matter and galaxy formation,” said Risa Wechsler, director of the Kavli Institute for Particle Astrophysics and Cosmology (KIPAC). Last year, for example, the DES team used data on satellite galaxies in conjunction with computer simulations to place much tighter limits on dark matter’s interactions with ordinary matter.
Now, the team is using data from a comprehensive search over most of the sky to ask different questions, including how much dark matter it takes to form a galaxy, how many satellite galaxies we should expect to find around the Milky Way and whether galaxies can bring their own satellites into orbit around our own – a key prediction of the most popular model of dark matter.
Hints of galactic hierarchy
The answer to that last question appears to be a resounding “yes.”
The possibility of detecting a hierarchy of satellite galaxies first arose some years back when DES detected more satellite galaxies in the vicinity of the Large Magellanic Cloud than they would have expected if those satellites were randomly distributed throughout the sky. More data was needed to conclusively attribute this excess to galaxies that arrived at the Milky Way with the Large Magellanic Cloud.
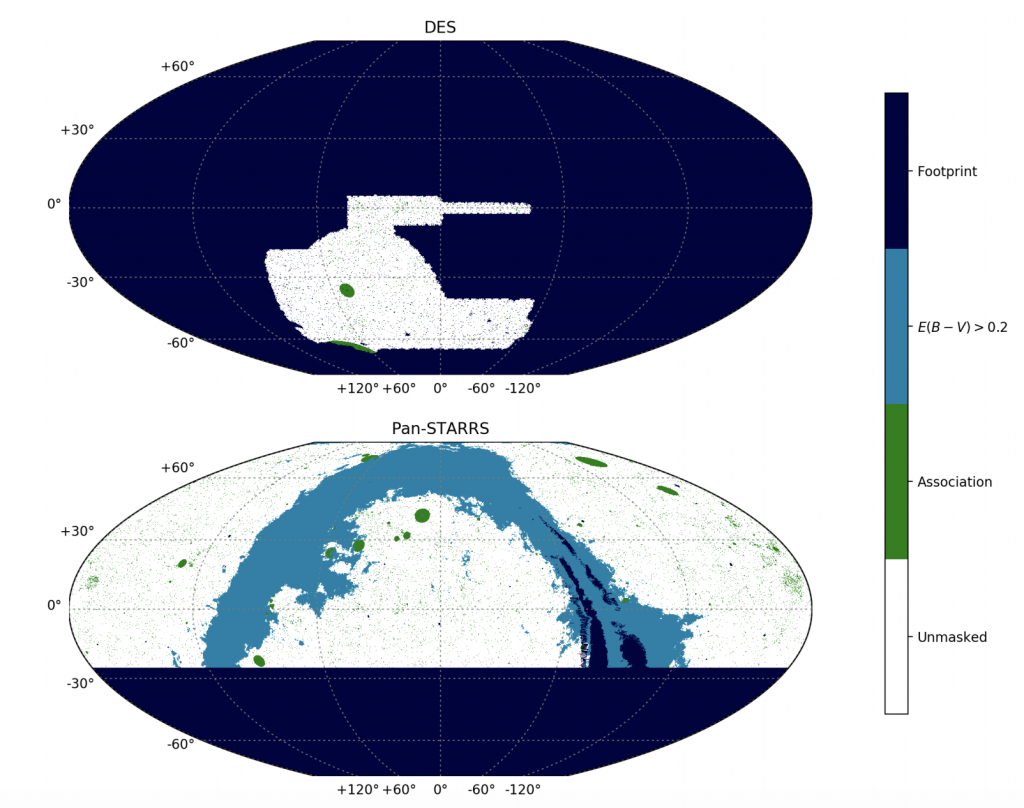
In the first published study, the DES group combined observations from DES with those from the Pan-STARRS survey, together covering 75% of the sky, to test this hypothesis. The DES data represents nearly 40,000 images from a 500-million-pixel camera collected over three years from a telescope in Chile.
The raw DES data was run through a series of data compressions, including a final step led by Bechtol’s group, to identify and catalog individual stars, including their color, which infers temperature, and how far away they are.
“We throw the star catalog into our search algorithms, which are responsible for identifying small groups of stars that are clustered in space and have similar colors and brightness. There’s a particular distribution for what we expect the stars to look like in ultrafaint galaxies,” says UW-Madison physics graduate student Mitch McNanna. “Even then we’re not 100 percent sure that we’ve found a real galaxy, so we also collect spectroscopic observations to measure the doppler motion of the stars. Hopefully we see the group of stars is moving in a way that’s different from the rest of the stars in the Milky Way halo.”
The team, including Alex Drlica-Wagner at Fermilab, produced a model of which satellite galaxies are most likely to be seen by current surveys, given where they are in the sky as well as their brightness, size and distance.
In the second study, led by others in the DES team including Ethan Nadler at Stanford University and collaborators, the team took the findings of the latest satellite census and analyzed computer simulations of millions of possible universes. Those simulations model the formation of dark matter structure that permeates the Milky Way, including details such as smaller dark matter clumps within the Milky Way that are expected to host satellite galaxies. To connect dark matter to galaxy formation, the researchers used a flexible model that allows them to account for uncertainties in the current understanding of galaxy formation, including the relationship between galaxies’ brightness and the mass of dark matter clumps within which they form.
Those components in hand, the team ran their model with a wide range of parameters and searched for simulations in which LMC-like objects fell into the gravitational pull of a Milky Way-like galaxy. By comparing those cases with galactic observations, they could infer a range of astrophysical parameters, including how many satellite galaxies should have tagged along with the LMC. The results were consistent with Gaia observations: Six satellite galaxies should currently be detected in the vicinity of the LMC, moving with roughly the right velocities and in roughly the same places as astronomers had previously observed. The simulations also suggested that the LMC first approached the Milky Way about 2.2 billion years ago, consistent with high-precision measurements of the motion of the LMC from the Hubble Space Telescope.
Galaxies yet unseen
In addition to the LMC findings, the team also put limits on the connection between dark matter halos and galaxy structure. For example, in simulations that most closely matched the history of the Milky Way and the LMC, the smallest galaxies astronomers could currently observe should have stars with a combined mass of around a hundred suns, and about a million times as much dark matter. According to an extrapolation of the model, the faintest galaxies that could ever be observed could form in halos up to a hundred times less massive than that.
And there could be more discoveries to come: If the simulations are correct, there are around 150 more satellite galaxies – more than double the number already discovered – hovering around the Milky Way. The discovery of those galaxies would help confirm the researchers’ model of the links between dark matter and galaxy formation, and likely place tighter constraints on the nature of dark matter itself.
Sarah McCarthy earns fellowship through National Science Foundation’s Graduate Research Fellowship Program
Congrats to first-year grad student Sarah McCarthy on being named a 2020 NSF GRFP recipient!
The Physics Learning Center goes online for remote learning
Like course instruction, The Physics Learning Center and other tutoring and advising groups on campus have gone online to support their students’ learning. PLC was featured in an article about how these Centers are adapting to instructional changes during the pandemic.