The Department of Physics is pleased to announce that Ke Fang, assistant professor of physics and WIPAC investigator, has received the inaugural Bernice Durand Faculty Fellowship. This Fellowship, given in honor of the late Professor Emerit of Physics, Bernice Durand, recognizes Fang’s major contributions to the analysis of data from the NASA Fermi satellite, the High Altitude Water Cherenkov (HAWC) telescope and IceCube, and for fundamental theoretical insights in their multimessenger context. Fang is a Sloan Fellow, has been awarded an NSF CAREER award, and is the spokesperson for the HAWC experiment.
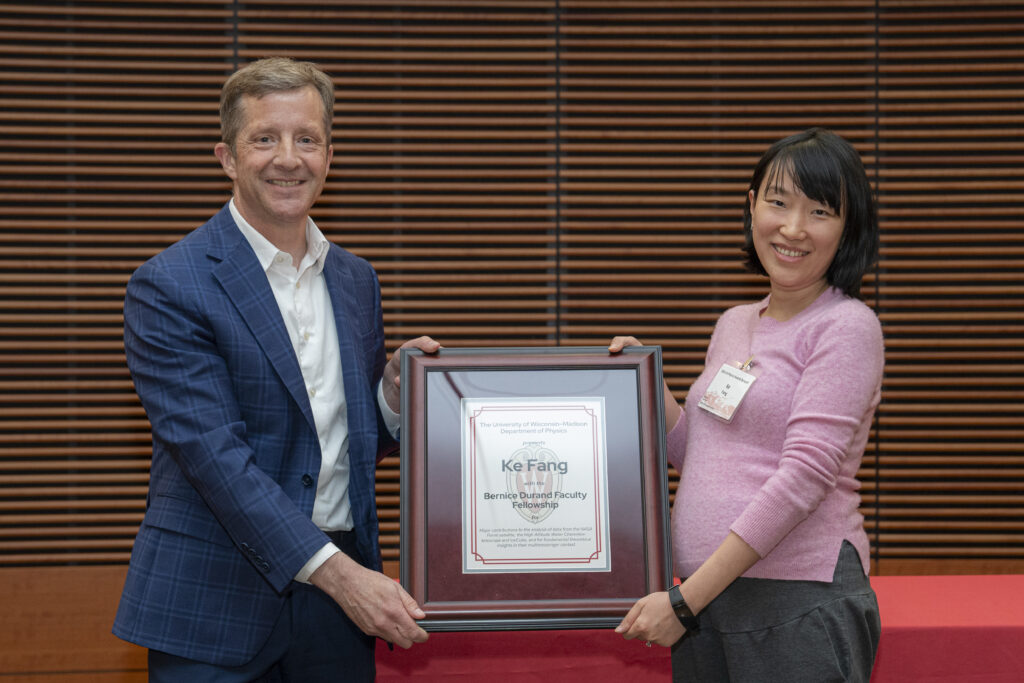
Durand was one of the first two women professors in the UW–Madison Department of Physics. While at UW–Madison, Durand was a theoretical physicist who specialized in particle theory and mathematical physics. Her research was on symmetry relations in algebra and physics, plus the phenomenology of high-energy interactions at large particle accelerators.
As the first Associate Vice Chancellor for Diversity & Climate, Professor Durand provided leadership to ensure that faculty, staff, and student diversity issues including race, ethnicity, gender, sexual preference, and classroom and general campus workplace climate issues be addressed, and that search committees for non-classified staff be trained in broadening the pool of applicants and eliminating implicit bias. Durand co-directed a grant from the Alfred P. Sloan Foundation to the UW System designed to create more equity, flexibility and career options for faculty and academic staff. She was also a member of the leadership team of the Women in Science and Engineering Leadership Institute sponsored by the National Science Foundation to increase the participation and status of women in science.
A recipient of the Chancellor’s Award for Outstanding Teaching, Professor Durand taught courses at all levels, from modern physics for non-scientists (“Physics for Poets”) to a specialized course she developed for advanced graduate students in the use of topology and algebra in quantum field theory. In the mid 1990s, she used technological and pedagogical techniques in her teaching, such as broadcasting her modern physics for non-scientists course on public television with web-based coursework, and pioneering one of two early versions of MOOCs (massive open online courses) on campus.
Durand passed away in 2022.
The Bernice Durand Faculty Fellowship was conceived by our Board of Visitors, who spearheaded the ultimately-successful fundraising effort, with support from Professor Emerit Randy Durand for this fellowship honoring his wife.