No atom is an island, and scientists have known for decades that groups of atoms form communities that “talk” to each other. But there is still much to learn about how atoms — particularly energetically excited ones — interact in groups.
In a study published in PRX Quantum, physicists from the University of Wisconsin–Madison observed communication between atoms at lower and lower densities. They found that the atoms influence each other at 100 times lower densities than probed before, exhibiting slow decay rates and emitting coherent light.
“It seems that (low-density) groups of excited atoms spontaneously organize to then produce light that is coherent,” says David Gold, a postdoctoral fellow in Deniz Yavuz’s group and lead author of the study. “These findings are pretty interesting from a basic science standpoint, and in terms of quantum computing, the takeaway is that even with very low numbers of atoms, you can see significant amounts of (these effects).”
A well-established property of atoms is found in electron excitation: when a specific wavelength of light hits an atom of a specific element, an electron is excited to a higher orbital level. As that electron decays back to its initial state, a photon of a specific wavelength is emitted. A single atom has a characteristic decay rate for that process. When groups of atoms are studied, their interactions are observed: the initial decay rate is very fast, or superradiant, then transitions to a slower, or subradiant, rate.
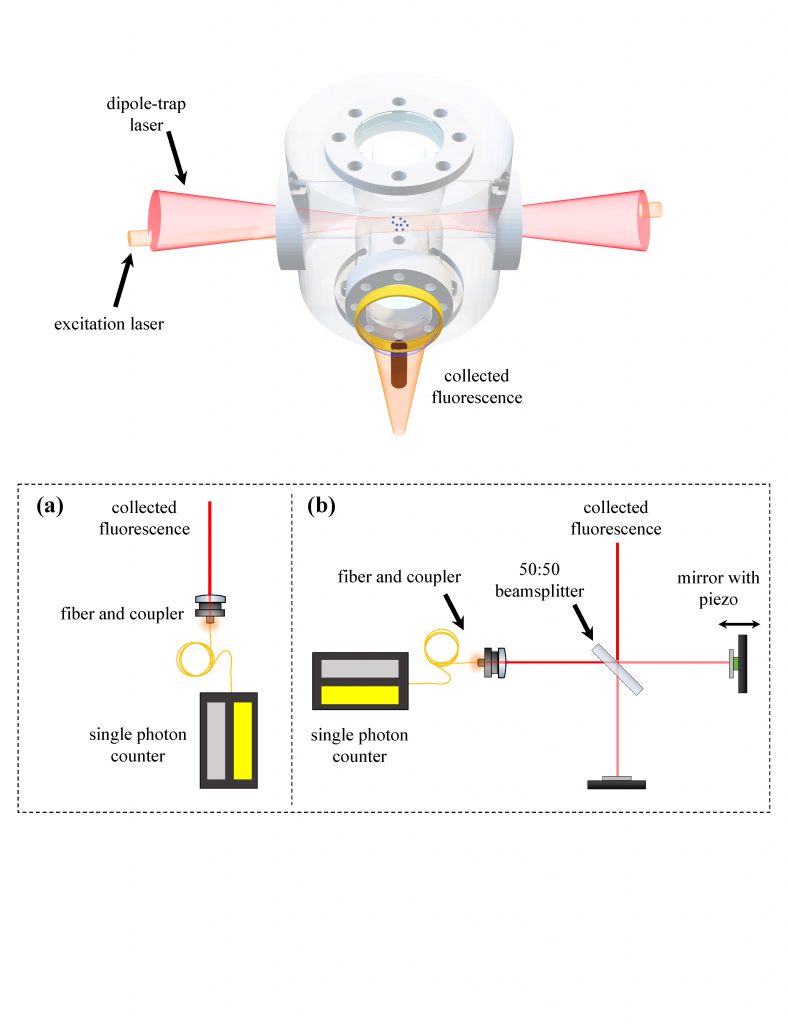
Though well-established in dense clouds, this group-talk has never been studied in less dense clouds of atoms, which could have impacts on applications such as quantum computing.
In their first set of experiments, Gold and colleagues asked what the decay rate of lower-density clouds looked like. They supercooled the atoms in a cloud, hit them with an excitation laser, and recorded the decay rates as an intensity of emitted light over time. They observed the characteristic subradiance. In this case, they did not always see superradiance, likely due to the reduced number of atoms available to measure.
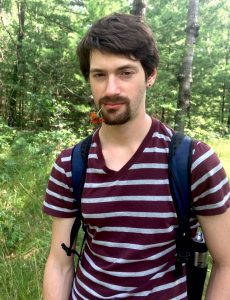
Next, they asked what happened if they let the cloud expand — or decrease in density — for varying periods of time before repeating their experiment. They found that as the cloud become less and less dense, the amount of subradiance decreased, until eventually a density was reached where the atoms stopped behaving like a group and instead displayed single-atom decay rates.
“The most subradiance that we observed was at around a hundred times lower optical density than it had previously been observed before,” Gold says.
Now that the researchers knew that a less dense cloud still decays subradiantly to a point, they asked if the decay was happening in an isolated manner, or if the atoms were really acting as a group. If acting as a group, the emitted light would be coherent, or more laser-like, with some structure between the atoms.
They used the same experimental setup but added an interferometer, where light is split and recombined before the photons are detected. They first set the baseline interference pattern by moving the mirror closer or further away from the splitter — changing the path length of one of the beams — and mapping the interference pattern of the split light waves that were emitted from the same atom.
If there were no relationship between the two atoms and the light they emit, then they would have expected to see no interference pattern. Instead, they saw that for some distance of mirror displacement, the lightwaves did interfere, indicating that different atoms being measured were nonetheless producing coherent light.
“I think this is the more exciting thing we found: that the light that’s being emitted is coherent and it has more of the properties of a laser than you would expect,” Gold says. “The atoms are influenced by each other and not in a way we would have expected.”
Aside from the interesting physics seen in the study, Gold says the work is also applicable to quantum computing, particularly as those computers grow bigger in the future.
“Even if everything in a quantum computer is running perfectly and the system was completely isolated, there’s still this inherent thing of, well, the atoms just might decay down from [the computational] state,” Gold says.
This work was supported by National Science Foundation (NSF) Grant No. 2016136 for the QLCI center Hybrid Quantum Architectures and Networks.