In the 27 years that Peter Timbie has been a UW–Madison physics professor, he has largely been able to conduct cutting-edge research in the field of the cosmic microwave background, or CMB, with just himself and a handful of graduate students or other researchers in his group.
“You can’t do that anymore,” Timbie says. “Now, CMB experiments require hundreds of people. I finally decided that I’m not going to be able to do this on my own anymore.”
Timbie’s CMB colleague, assistant professor of physics Moritz Münchmeyer, joined the UW–Madison faculty in 2021 as a member of one of those large experiments: Simons Observatory. But his membership was a carryover from his time as a post-doc, and so he lost full access once he became an independent investigator.
“Simons Observatory is the big new CMB experiment that is upcoming, and it will push the field of CMB science forward,” Münchmeyer says. “For us to do state-of-the-art CMB science in the next five to ten years, and to set us up for the future, being in Simons Observatory is very important.”
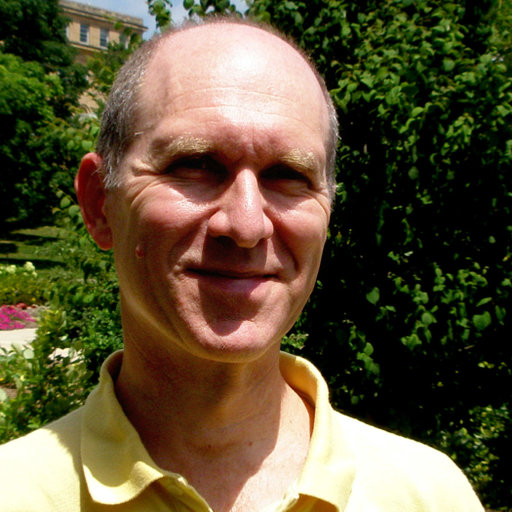
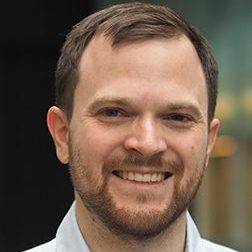
In early 2024, Timbie and Münchmeyer were accepted as institutional members of Simons Observatory, granting them and their research groups full access to the collaboration of scientists and the wealth of data that Simons offers.
Simons Observatory is currently being built in the desert of the Chilean Andes and is expected to begin operations later this year. Its main goal is the detection of primordial gravitational waves — those from the Big Bang that have never been detected before.
“Primordial gravitational waves are expected to be produced by quantum mechanical effects in the early universe. This would be the first time that we observe direct evidence for quantum perturbations of space-time,” Münchmeyer says. “But it’s not known how large these waves are, so Simons Observatory could find them — which would be sensational news — otherwise, it will set tighter boundaries and exclude a lot of models.”
Münchmeyer, Timbie and their research groups would now be collaborators on this gravitational waves research, but they also have other interests in the CMB field that membership will help them address. CMB is radiation that comes from around 300,000 years after the Big Bang and is currently the earliest light in the universe that scientists can detect. CMB telescopes, including Simons, detect light in the microwave range and measure tiny temperature and polarization fluctuations at temperatures just a few degrees above absolute zero.
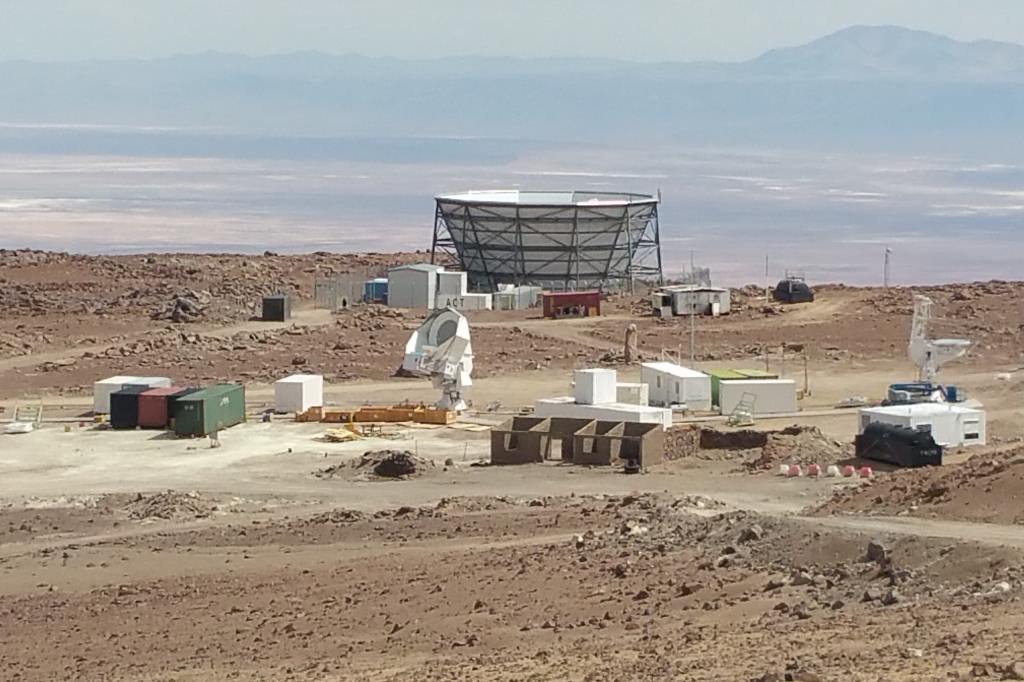
“CMB telescopes probe all the matter that this radiation encountered on its way from the Big Bang to us,” Münchmeyer says. “CMB light is scattering on charges in the universe, and then these interactions induce a change in temperature that we can measure.”
Münchmeyer and his group plan to use the new, more powerful Simons data to study two problems in CMB. First, the Simons data should be powerful enough to measure the kinetic Sunyaev–Zeldovich, or KSZ, effect, which he can use to measure the velocity field of the universe and probe properties of the primordial perturbations. Second, he plans to constrain models of inflation, which refers to the earliest times of the universe, before the so-called “hot” Big Bang.
“Inflation is where I started in cosmology ten years ago, and now Simons Observatory will have better data,” Münchmeyer says. “The interesting thing about inflation is that it happened at an energy scale that is far higher than the one a particle collider could probe, so it can probe the energy scales of grand unified theories or perhaps even quantum gravity, if a signal is found.”
Timbie, whose expertise lies in instrumentation and detector building, is looking forward to contributing to expansions of the Observatory that are already being planned. He also sees an opportunity for combining upcoming data from Simons with data from instruments he has helped design and build that study the universe closer to the present day.
“As Moritz mentioned, as the CMB travels to us from near the Big Bang, it’s distorted, but you can’t easily tell at what point during the excursion that those photons were disturbed,” Timbie says. “I’ve been working on a different experiment which is very good at mapping the universe in different slices heading back towards the Big Bang. And I think there’s a way to combine these datasets to add some information to the CMB data that would give us a very powerful tool for making large, three-dimensional maps of the Universe.”
Münchmeyer and Timbie certainly have the CMB expertise to have easily been accepted as Simons members, but membership also requires a fairly significant buy-in, which was fully supported by the UW–Madison department of physics, the Office of the Vice Chancellor for Research, and the College of Letters & Science. Their physics colleagues were instrumental in securing their membership.
“Our colleagues from accelerator physics, for example, were very supportive and pointed out that in the early days, before anyone was involved in the ATLAS or CMS experiments, the University had to buy into those collaborations,” Timbie says. “And of course, the payoff from that has been unbelievably large. The hope is maybe something similar will happen for us with the big CMB experiments.”
Of this potential, Münchmeyer adds: “After Simons Observatory, there will be the so-called CMB S4 experiment, which is the highest-ranked experiment in the new P5 Particle Physics report. The Department of Energy is very heavily investing in CMB physics.”
Simons Observatory’s Badger connections
Brian Keating, Timbie’s second graduate student, is the director of Simons Observatory; the Simons principal investigator, Mark Devlin ’88, worked with Prof. Dan McCammon as an undergraduate physics major